Cryptosporidium parvum: an emerging pathogen
by Greg Hannahs, Kenyon College
Introduction
Life Cycle
Clinical Manifestations
Epidemiology
Transmission
Pathogenesis
Detection and Diagnosis
Treatment
Drinking water: purification and filtration
Recent Progress and Future Directions
Cryptosporidium is a coccidian protozoan parasite that has gained much attention in the last 20 years as a clinically important human pathogen. The discovery of Cryptosporidium is usually associated with E.E. Tyzzer, who, in 1907, described a cell-associated organism in the gastric mucosa of mice (Keusch, et al., 1995). For several decades, Cryptosporidium was thought to be a rare, opportunistic animal pathogen, but the first case of human cryptosporidiosis in 1976 involved a 3-year-old girl from rural Tennessee who suffered severe gastroenteritis for two weeks (Flanigan and Soave, 1993). Electron microscopic examination of the intestinal mucosa led to the discovery that Cryptosporidium parvum was the infectious species in humans. In the early 1980s, the strong association between cases of cryptosporidiosis and immunodeficient individuals (such as those with AIDS--acquired immunodeficiency syndrome) brought Cryptosporidium to the forefront as a ubiquitous human pathogen. Presently, the increasing population of immunocompromised persons and the various outbreaks of cryptosporidiosis through infection by water-borne Cryptosporidium oocysts (often in drinking water) have placed an even greater emphasis on this pathogen. Little is known about the pathogenesis of the parasite, and no safe and effective treatment has been successfully developed to combat cryptosporidiosis (Juranek, 1995). Unlike other intestinal pathogens, Cryptosporidium can infect several different hosts, can survive most environments for long periods of time due to its "hardy cyst" (Keusch, et al., 1995), and inhabits all climates and locales. Below is a duodenal biopsy sample from a patient with AIDS and cryptosporidiosis (Flanigan and Soave, 1993; arrows point to the parasites in the microvillus border):
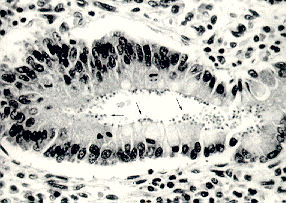
Cryptosporidium is taxonomically classified as a Sporozoa, since its oocyst releases four sporozoites (its motile infectious agents) upon excystation. However, it differs from related parasites such as Toxoplasma by its monoxenous life cycle--completing its entire cycle within a single host (Flanigan and Soave, 1993). The life cycle is complex; there are both sexual and asexual cycles, and there are six distinct developmental stages (Keusch, et al., 1995):
- Excystation of the orally ingested oocyst in the small bowel with release of the four sporozoites
- Invastion of intestinal epithelial cells via the differentiated apical end of the sporozoite within a vacuole formed of both host and parasite membranes and the initiation of the asexual intracellular multiplication stage
- Differentiation of microgametes and macrogametes
- Fertilization initiating sexual replication
- Development of oocysts
- The formation of new, infectious sporozoites within the oocyst, which is then excreted in the stool
The cycle begins anew when these oocysts are ingested by a new host. Below is a visual representation of the Cryptosporidium life cycle (Heyworth, 1992):
The various symptoms of cryptosporidiosis differ greatly between immunocompetent and immunocompromised individuals. In immunocompetent patients, cryptosporidiosis is an acute, yet self-limiting diarrheal illness (1-2 week duration), and symptoms include (Juranek, 1995):
- Frequent, watery diarrhea
- Nausea
- Vomiting
- Abdominal cramps
- Low-grade fever
For immunocompromised persons, the illness is much more severe (Juranek, 1995):
- Debilitating, cholera-like diarrhea (up to 20 liters/day)
- Severe abdominal cramps
- Malaise
- Low-grade fever
- Weight loss
- Anorexia
Due to lack of tissue specificity, C. parvum infection has also been identified in the biliary tract (causing thickening of the gallbladder wall) and the respiratory system (Casemore, et al., 1994).
Infection by C. parvum has been reported in six continents and identified in patients aged 3 days to 95 years old (Flanigan and Soave, 1993). Transmission is usually fecal-oral, often through water contaminated by livestock mammal feces. Persons most likely to be infected by Cryptosporidium are:
- infants and younger children in day-care centers
- those whose drinking water is unfiltered and untreated
- involved in farming practices such as lambing, calving, and muck-spreading
- engaging in sexual practices that brings a person into oral contact with feces of an infected individual
- patients in a nosocomial setting with other infected patients or health-care employees
- veterinarians who come in contact with farm animals
- travelers to areas with untreated water
- living in densely populated urban areas
- owners of infected household pets (rare)
(Keusch, et al., 1995; Casemore, et al., 1994; Goodgame, 1996; Juranek, 1995; Flanigan and Soave, 1993)
Within a population of immunocompromised individuals, severe and persistent disease has been associated with persons with CD4 counts of <180 cell/cubic mm (Juranek, 1995).
Cryptosporidial infection can thus be transmitted from fecally contaminated food and water, from animal-person contact, and via person-person contact. The probability of transmission from just a small amount of contamination is fairly high, since a recent study has determined that the 50% infective dose (ID50) of C. parvum is only 132 oocysts for healthy persons with no previous serological immunity to cryptosporidiosis (DuPont, et al., 1995).
Food and water
There have been six major outbreaks of cryptosporidiosis in the United States as a result of contamination of drinking water (Juranek, 1995). One major outbreak in Milwaukee in 1993 affected over 400,000 persons. Outbreaks such as these usually result from drinking water taken from surface water sources such as lakes and rivers (Juranek, 1995). Swimming pools and water park wave pools have also been associated with outbreaks of cryptosporidiosis. Also, untreated groundwater or wellwater public drinking water supplies can be sources of contamination.
The highly environmentally resistant cyst of C. parvum allows the pathogen to survive various drinking water filtrations and chemical treatments such as chlorination. Although municipal drinking water utilities may meet federal standards for safety and quality of drinking water, complete protection from cryptosporidial infection is not guaranteed. In fact, all waterborne outbreaks of cryptosporidiosis have occurred in commmunities where the local utilities met all state and federal drinking water standards (Juranek, 1995). For more information about drinking water and cryptosporidiosis, click here.
Food can also be a source of transmission, when either an infected person or an asymptomatic carrier contaminates a food supply. The first documentation of this type of infection occurred at a county fair in Maine, where children who drank apple cider contaminated by animal feces developed cryptosporidiosis (Juranek, 1995). The oocysts do not survive cooking, but food contamination can occur in beverages, salads, or other foods not heated or cooked after handling.
Animal-person transmission
Transmission of C. parvum from household pets is extremely rare, but there is a definite correlation between calves and humans--approximately 50% of calves shed oocysts and the pathogen is present on upwards of 90% of all dairy farms (Juranek, 1995).
Person-person transmission
Cryptosporidium transmission occurs at a high frequency in day-care centers, where infants or younger children are clustered within classrooms, share toilets and common play areas, or necessitate frequent diaper-changing (Keusch, et al., 1995). Day-care employees can become easily infected by C. parvum through careless diaper-changing or through washing the laundry of infected children. Day-care workers can then spread the pathogen to their families at home.
Nosocomial settings are also a major forum for cryptosporidial transmission. There have been several reports of both transmission from patients to health care staff and patient-to-patient transmission. An outbreak in a bone-marrow transplant unit occurred where five patients developed cryptosporidiosis after a sixth, infected patient was admitted to the unit (Casemore, et al., 1994). Another major outbreak occurred in North Wales after a terminally ill AIDS patient infected with cryptosporidiosis was admitted to the infectious diseases unit of a major hospital. Five cases of cryptosporidiosis were confirmed among the nursing staff, and infection most likely occurred from major environmental contamination due to the patient's intractable diarrhea and vomiting (Casemore, et al., 1994). This "environmental contamination" raises the possibility of aerosol transmission of C. parvum from person-to-person. Various routes of transmission such as aerosol infection is fairly likely, since Cryptosporidium oocysts are shed in large numbers during acute infection and are immediately infective to others (Casemore, et al., 1994). These nosocomial outbreaks signal the need for hospitals to take extreme enteric precautions upon admittance of infected patients. And, health-care staff should report even minor gastrointestinal symptoms to prevent transmission to other staff and patients.
Upon oocyst excystation, four sporozoites are released which adhere their apical ends to the surface of the intestinal mucosa (Keusch, et al., 1995). Below is a phase contrast photograph of sporozoite release from the Cryptosporidium oocyst (Flanigan and Soave, 1993):
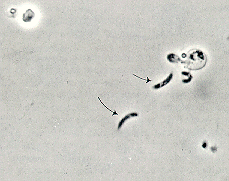
A sporozoite-specific lectin adherence factor has been identified as the agent of attachment to the intestinal surface (Keusch, et al., 1995). After sporozoite attachment, it has been hypothesized that the epithelial mucosa cells release cytokines that activate resident phagocytes (Goodgame, 1996). These activated cells release soluble factors that increase intestinal secretion of water and chloride and also inhibit absorption. These soluble factors include histamine, serotonin, adenosine, prostaglandins, leukotrienes, and platelet-activating factor, and they act on various substrates, including enteric nerves and on the epithelial cells themselves (Goodgame, 1996). Consequently, epithelial cells are damaged by one of two models:
- Cell death is a direct result of parasite invasion, multiplication, and extrusion or
- Cell damage could occur through T cell-mediated inflammation, producing villus atrophy and crypt hyperplasia
Either model produces distortion of villus architecture and is accompanied by nutrient malabsorption and diarrhea (Goodgame, 1996). Experimental evidence supporting this pathogenic hypothesis exists in a pig model system, where decreased intestinal sodium absorption has been correlated with "both decreased villus surface area and inhibition by prostaglandin E2 produced by inflammatory cells" (Goodgame, 1996).
When C. parvum was first identified as a human pathogen, diagnosis was made by a biopsy of intestinal tissue (Keusch, et al., 1995). However, this method of testing can give false negatives due the "patchy" nature of the intestinal parasitic infection (Flanigan and Soave, 1993). Staining methods were then developed to detect and identify the oocysts directly from stool samples. The modified acid-fast stain is traditionally used to most reliably and specifically detect the presence of cryptosporidial oocysts (image from Ortega, et al., 1993):
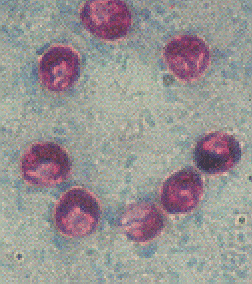
Immunologically, anti-cryptosporidial IgM, IgG, and IgA can be detected by the enzyme-linked immunoabsorbent assay (ELISA) or by the antibody immunofluorescence assay (IFA), but neither of these assays can provide a direct diagnosis of cryptosporidiosis.
Recently, new genetic methods of detecting C. parvum have been developed, using PCR (Polymerase Chain Reaction) or other DNA-based detection methods. For more information about these improvements in the specificity and sensitivity of cryptosporidial detection, click here.
No safe and effective therapy for cryptosporidial enteritis has been successfully developed. Since cryptosporidiosis is a self-limiting illness in immunocompetent individuals, general, supportive care is the only treatment for the illness. Oral or intravenous rehydration and replacement of electrolytes may be necessary for particularly voluminous, watery diarrhea. Oral rehydration treatment can include Gatorade, bouillon, or oral rehydration solution, containing glucose, sodium bicarbonate, and potassium (Flanigan and Soave, 1993).
Pharmocological therapies
For immunocompromised patients with cryptosporidiosis, several antimicrobial agents have been tested as possible treatments for the illness. Antibiotics such as spiramycin and dicalzuril sodium have produced partial responses in patients (a partial decrease in diarrhea or partial decrease in stool oocyst number), but have not yielded reliable, reproducible results (Flanigan and Soave, 1993). However, one particular antimicrobial agent, paromomycin, has been shown to decrease the intensity of infection and improve intestinal function and morphology (Goodgame, 1996). Paromomycin is a poorly absorbed, broad spectrum antibiotic similar to neomycin, and in a review of 12 patients with 23 episodes of gastrointestinal cryptosporidiosis, 16 of the 23 epidoses had a complete response to therapy (symptom improvement, diarrhea eradication, and weight gain) and the other seven episodes had a partial response (Flanigan and Soave, 1993). However, relapse after treatment is common and fairly expensive maintenance therapy is often necessary.
Immunologic therapy
Although serological antibodies do not provide protection from cryptosporidial infection, several studies have been done to show that antibodies in the intestinal lumen may help clear or even prevent infection. The feeding of bovine colostral immunoglobulin to patients has been shown to ameliorate symptoms of Cryptosporidium infection in humans (Heyworth, 1992), and it has also been shown that the release of intestinal IgA accompanies this clearance of infection. In addition, anti-sporozoite antibodies have blocked the infectivity of C. parvum sporozoites in mice by inhibiting their ability to attach to the surface of the intestininal mucosa. A more recent study by Doyle, et al. (1993), reproduced the inhibition of C. parvum infection by hyperimmune bovine colostrum in vitro, providing an ideal system through which cryptosporidial infection can be studied in the laboratory. The mean number of intracellular parasites per host cell was reduced by 61% upon introducing HBC Ig antibodies with a concentration of 1 mg/ml IgG. This investigation also purified antibodies from HBC Ig on Western blots of Cryptosporidium proteins and found that they, too, inhibited C. parvum infectivity in vitro. Lastly, this team of investigators identified 19 sporozoite surface antigens which are recognized by HBC Ig. This system of immunotherapy (intraluminal gastric administration of HBC to immunocompromised patients) is a big step forward in finding an efficacious treatment for cryptosporidiosis. However, a combination of pharmacologic and immunologic therapy may be the most effective scenario for combating C. parvum infection in immunodeficient persons.
Home use
Current data is not adequate to advise all immunodeficient persons to boil or avoid tap water, but the risks involved are high enough that, until the health risk of drinking water containing small number of Cryptosporidium oocysts is clearly defined, it is advised that these individuals boil all water intended for drinking for at least one minute.
If one does not want to boil water constantly, a household water filtration system or drinking bottled water can reduce the risk of Cryptosporidium infection. When selecting a filtration system, the system should have one or all of the following characteristics (Juranek, 1995):
- it can remove particles that are 0.1-1 micrometers in size
- filters water by reverse osmosis
- it has an "absolute" 1-micron filter
- meets NSF standard no. 53 for "cyst removal"
On the other hand, filters that have the following characteristics do NOT guarantee >99% removal of Cryptosporidium oocysts (Juranek, 1995):
- filters with a "nominal" 1-micron rating
- only employs ultraviolet light
- uses only activated carbon for filtration
- utilizes pentiodide-impregnated resins
- it is effective against Giardia species
When selecting a high-quality bottled water, one should question the vendor directly to see if the filtration systems used to purify the bottled water matches the characteristics in the first list above (removal of >99% oocysts). In either case, careful research is needed to ensure protection from Cryptosporidium infection from drinking water at home, especially for immunocompromised individuals.
Municipal systems
Municipal water utilities provide relatively good protection against water-borne Cryptosporidium infection. There were only six major documented cases of cryptosporidiosis outbreaks via drinking water between 1984-1994, even though the 1993 outbreak in Milwaukee caused over 400,000 cases (Jakubowski, 1995). Since this massive outbreak, much more research has been done to eliminate the possibility of further outbreaks via public drinking water.
Municipal drinking water is purified two ways: through chemical treatments and through filtration. Chemically, chlorination is used most frequently to safely disinfect drinking water by killing most viruses, bacteria, and protozoa like Giardia, but studies have shown that Cryptosporidium is 240,000 times more resistant to chlorination than Giardia (Jakubowski, 1995), and C. parvum oocyst viability was not affected by exposure to 1.05 and 3% chlorine for up to 18 hours (Korich, et al., 1990). Also, Korich, et al., found that chlorine dioxide and monochloramine were also ineffective in inactivating C. parvum oocysts in drinking water. Excystation and mouse infectivity were evaluated to assess oocyst viability. This study did find that treating oocysts with 1 ppm (1 mg/liter) of ozone for five minutes inactivated over 90% of the oocysts, but drinking water standards dictate that a maximum of only 0.4 mg of ozone per liter of water is allowed (Juranek, 1995). Thus, although ozone may be a potential disinfectant for inactivating C. parvum oocysts, one should not assume that these traditional chemical disinfectants (even if they inactivate coliform bacteria and Giardia) effectively eliminate the risk of cryptosporidial infection.
Filtration is a better bet for removing C. parvum oocysts from municipal drinking water. In recent years, ultra-fine membranes have been developed to remove various contaminants from drinking water. Below is a typical membrane process unit from Mount Vernon, Ohio (five miles from Kenyon College!):
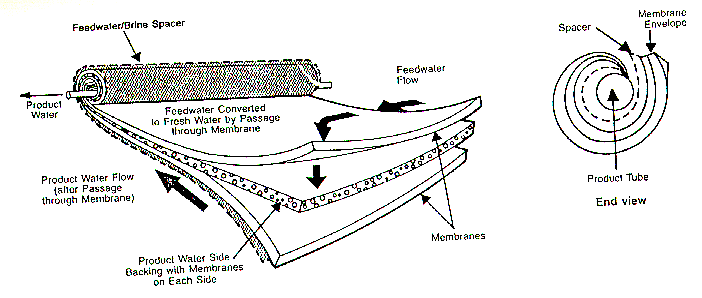
The following is the removal size ranges of membrane processes (1 micron = .0000394 inches):
- 5-100 microns: conventional filtration, removes human hair, the smallest particles visible to the naked eye and red blood cells
- 0.1-5 microns: micro filtration, removes the smallest yeast cells, tobacco smoke, and the smallest bacteria
- 0.01-0.1 microns: ultra filtration, removes carbon black and is in the range of an electron microscope
- 0.001-0.01 microns: reverse osmosis, removes particles in the ionic range, such as the polio virus, aqueous salts, and metal ions (Camp Dresser and McKee, 1995)
Thus, since C. parvum oocysts can be as small as 4 microns (Flanigan and Soave, 1993), at least micro filtration is needed to reliably remove oocysts from the water supply. Since Cryptosporidium oocysts are so resistant to chemical disinfectants, ultra filtration or reverse osmosis would provide ideal protection against waterborne outbreaks via drinking water.
Genetic methods for detecting oocysts have been developed that identify and amplify Cryptosporidium nucleic acids using the Polymerase Chain Reaction (Johnson, et al., 1995). Oocysts were detected by PCR in wastewater, surface waters, and drinking water, but the sensitivity of the PCR assay was inhibited by "uncharacterized components in the samples." The study went on to find that flow cytometry, dot blot, and magnetic antibody capture improved the sensitivity of the PCR assay.
A month later, in December of 1995, a test was developed that not only specifically and sensitively detected C. parvum oocysts by PCR (it amplified an 873-bp region of a 2359-bp DNA fragment encoding a repetitive oocyst protein), it reported the oocysts' viability (Wagner-Wiening and Kimmig, 1995). Direct use of PCR did not distinguish between live and dead oocysts, since oocyst DNA is apparently preserved at least a week after cell death. However, PCR was then used to detect target DNA of excysted sporozoites after incubation in excystation medium. Thus, viability of oocysts was determined by detecting and amplifying a viable sporozoite DNA fragment. These protocols are adequate to detect low numbers of viable oocysts, such as during "routine monitoring of drinking water and environmental samples" (Wagner-Wiening and Kimmig, 1995).
Future directions
Since Cryptosporidium has the potential to infect many people from a point-source outbreak, much research still needs to be done to (adapted from Juranek, 1995 and Keusch, et al., 1995):
- clarify the relationship between low numbers of oocysts in drinking water and the frequency of cryptosporidial infection
- determine the asymptomatic carrier rate for Cryptosporidium in immunocompromised persons and the chance that these individuals will develop cryptosporidiosis when their CD4 counts drop to a low level
- calculate the relative risks of infection from drinking water, contact with animals, unsafe sexual practices, and nosocomial contact to see where focus on preventative strategies should be placed
- improve state and federal communication for reporting cases of cryptosporidiosis and identifying outbreaks
- continue to develop more effective therapies for ameliorating cryptosporidiosis symptoms
Other Related Sites
Center For Disease Control
Cryptosporidium research at the Kansas State University
FDA public announcement
"Infectious cases of the month" at the University of Michigan
REFERENCES
Camp, Dresser, and McKee. "Summary of the Mt. Vernon, Ohio, Membrane Softening Pilot Plant." December 14, 1995.
Casemore, D.P., Garder, C.A., and O'Mahony, C. "Cryptosporidial infection, with special reference to nosocomial transmission of Cryptosporidium parvum: a review." Folia Parasitol, 1994; 41 (1): 17-21.
Doyle, P.S., Crabb, J., and Petersen, C. "Anti-Cryptosporidium parvum antibodies inhibit infectivity in vitro and in vivo." Infect Immun, 1993 Oct; 61 (10): 4079-84.
Flanigan, T.P. and Soave, R. "Cryptosporidiosis." Prog Clin Parasitol, 1993; 1-20.
Goodgame, R.W. "Understanding intestinal spore-forming protozoa: cryptosporidia, microsporidia, isospora, and cyclospora." Ann Intern Med, 1996 Feb 15; 124 (4): 429-41.
Heyworth, M.F. "Immunology of Giardia and Cryptosporidium infections." J Infect Dis, 1992 Sep; 166 (3): 465-72.
Jakubowski, W. "Giardia and Cryptosporidium: The Details." 1995 Safe Drinking Water Act Seminar, U.S. Environmental Protection Agency.
Johnson, D.W., Pieniazek, N.J., Griffin, D.W., Misener, L., and Rose, J.B. "Development of a PCR protocol for sensitive detection of Cryptosporidium oocysts in water samples." Appl Environ Microbiol, 1995 Nov; 61 (11): 3849-55.
Juranek, D.D. "Cryptosporidiosis: sources of infection and guidelines for prevention." Clin Infect Dis, 1995 Aug; 21 Suppl 1: S57-61.
Keusch, G.T., Hamer, D., Joe, A., Kelley, M., Griffiths, J., and Ward, H. "Cryptosporidia--who is at risk?" Schweiz Med Wochenschr, 1995 May 6; 125 (18): 899-908.
Korich, D.G., Mead, J.R., Madore, M.S., Sinclair, N.A., and Sterling, C.R. "Effects of ozone, chlorine dioxide, chlorine, and monochlorine on Cryptosporidium parvum oocyst viability." Appl Envion Microbiol, 1990 May; 56 (5): 1423-8.
Wagner-Wiening, C., and Kimmig, P. "Detection of viable Cryptosporidium parvum oocysts by PCR." Appl Environ Microbiol, 1995 Dec; 61 (12): 4514-6.
Brought to you by Hannahs Inc. productions