AHR and its Activating
Molecule ARNT Heterodimer: a Transcription Factor's Response to
TCDD
Jeremy Kauffman '21 and Ryan Nader '21
Contents:
I. Introduction
Functioning through the meditation of specific metabolic
pathways and environmental pollutants, the ligand-activated aryl
hydrocarbon receptor (AHR)
is responsible for
acting in a multitude of biological processes. Through multiple gene
regulation pathways, AHR
has been found to take part in the development of mammalian growth
rate, liver function, immune system development,and female conception
and lactation. AHR
also plays a role in drug metabolism and detoxification of toxic
combustion products such as polycyclic aromatic hydrocarbons (PAHs)
and aromatic amines. AHR
recognizes and binds ligands of varying nature within the cell and
allows for translocation into the nucleus, where it then forms a
heterodimer with the AHR
nuclear translocator (ARNT)
and interacts with a
specific sequence of DNA, allowing for the expression of target genes.
Based on the specific ligand bound to the AHR,
a variety of biological consequences may occur. More specifically, AHR
has been studied extensively in regard to the recognition and binding
of 2,3,7,8-Tetrachlorodibenzo-p-dioxin (TCDD). Once AHR
binds the TCDD ligand, translocation of both AHR
and the ligand into the nucleus may take place. This allows for the
mediation of the TCDD carcinogen pathway. Following translocation into
the nucleus, AHR
forms a heterodimer with ARNT.
The AHR-ARNT-TCDD
complex within the nucleus binds to a genomic sequence known as the
dioxin response element (DRE), expressing target genes. The expression
of these genes, including genes such as cytochrome P450 1A1, cause the
adverse effects associated with these highly carcinogenic dioxin
pollutants. Despite our knowledge of AHR�s
function specifically in regard to TCDD, other various ligand-bound AHR
regulatory roles remain relatively unexplored.
II. Mechanism
After ligand-bound AHR
dissociates its chaperone proteins that keep it dormant within the
cytoplasm, it translocates into the nucleus to form a heterodimer
with ARNT.
Although, if the AHR
repressor (AHRR) is present within a cell, it will bind ARNT
instead and repress AHR
from forming its heterodimer. This repression is done not through
competition, but rather a process known as transrepression, which
functions through protein-protein interactions that restrict AHR
heterodimerization.
If AHRR is not present in a cell, TCDD will bind the PAS B domain
of AHR
and the AHR-ARNT
heterodimer will form. Following the formation of the AHR-ARNT-TCDD
complex, the ligand-bound heterodimer will attach to the target
DNA known as the dioxin response element (DRE) and the heterodimer
will act as an activator that will induce increased expression of
target genes within the DRE, forming proteins such as CYP1A1. CYP1A1, which belongs to the
Cytochrome p450 family of enzyme , are found in most animal cells,
functioning in the metabolism of endogenous substances and the
detoxification of pollutants within the organism. Although,
when expression is largely upregulated with the activation
of the ligand-bound transcriptional factor AHR by TCDD,
these proteins catalyze harmful carcinogenic effects which
include malignant tumor initiation and promotion.
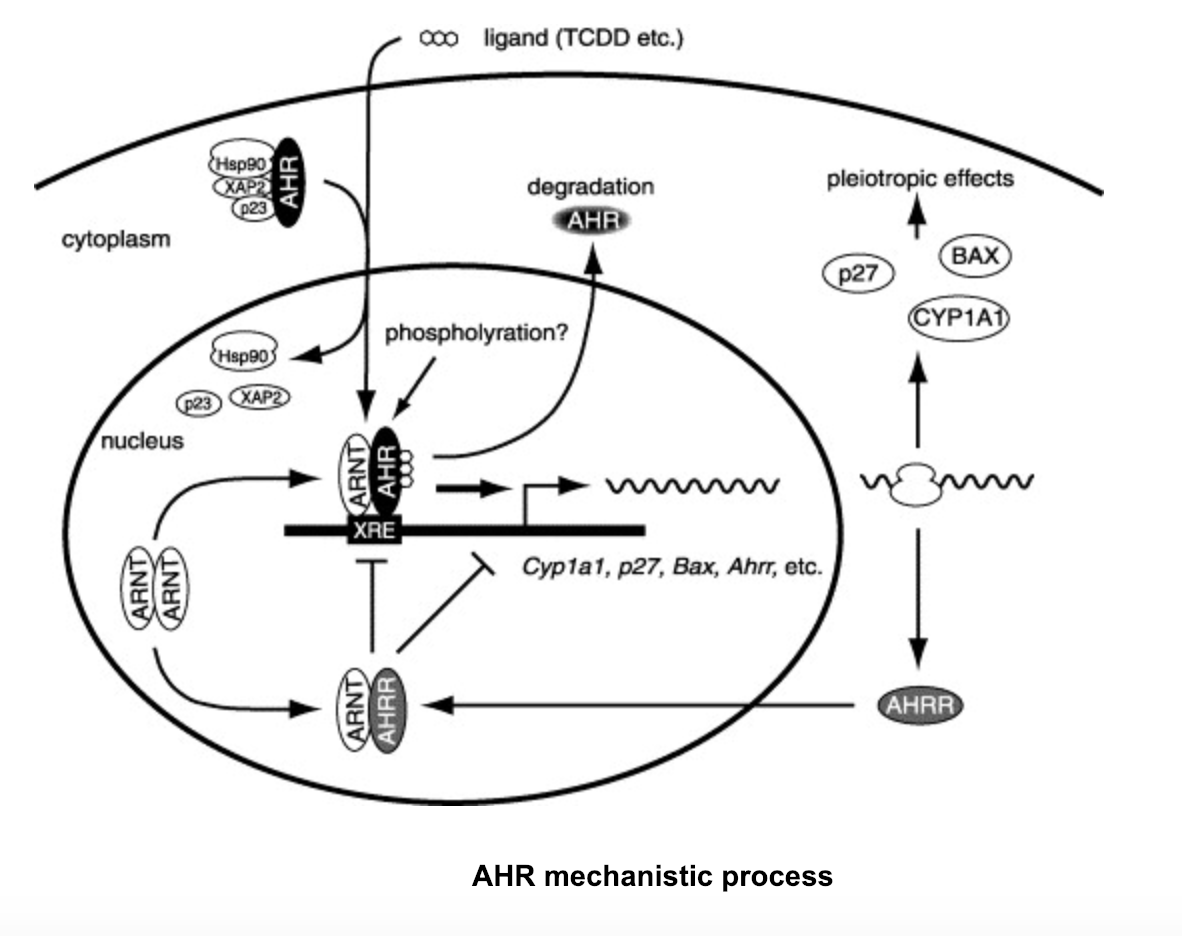 |
III. General Structure
AHR
and its activator protein ARNT
both belong to the PER-ARNT-SIM (PAS)/basic helix-loop-helix
(bHLH) family of transcriptional factors. The PAS domains, which
are comprised of two imperfect 50 amino acid repeats known as PAS
A and PAS B, are a highly conserved binding motif in which
protein-protein interactions or protein signaling can occur. The
PAS domain consists of a five-stranded antiparallel beta sheet and
multiple alpha helices that make up the hydrophobic core, allowing
both AHR
and ARNT
to interact
with each other and ligands. The basic helix-loop-helix (bHLH)
binding motif, which is found near the N-terminal region of AHR
and ARNT,
are binding arrangements that consist of two connected alpha
helices and aid in the interactions of protein-DNA and
protein-protein hetero/homodimerization. PAS-A domains aid in AHR-ARNT
heterodimer formation, and the bHLH of both AHR
and ARNT
allow for DNA binding with the heterodimer.
The PAS B domain of AHR,
also known as the ligand binding domain (LBD), specifically binds
to various ligands, allowing for a conformational change in AHR.
This conformational change removes the allosterically inhibiting
chaperone proteins, Hsp90, P23, and ARA9 that keep AHR
dormant in the cytoplasm. Once these proteins dissociate and the
ligand binds, the AHR-ligand
complex is able to translocate into the nucleus.
After entering the nucleus, ARNT
wraps around AHR
in a largely intertwined asymmetric construction. AHR-ARNT
dimerization is partially governed by the largely hydrophobic
points of contact to the dimerization interfaces between AHR
and ARNT
.
The heterodimer contains up to 35 hydrophobic residues that help
dimerization occur, as well as increase stability of the
heterodimer and its interaction with the DRE. The bHLH domains of
AHR
and ARNT
orient to form two similar protein extensions,
one
from AHR
and one
from ARNT,
that interact with the major groove of the DRE. Sequence-specific
interactions occur here as well, recognizing specifically the DRE
consensus sequence T
T G
C G
T G
and
differentiating it from extremely similar response elements
through interactions with the bHLH protein extensions found on
both the AHR
and ARNT
DNA binding sites.
AHR-ARNT Structure Formation and It's Interaction with the DRE
IV. Recognition of and Interaction with the Dioxin
Response Element
The two N-terminal bHLH protein extensions that interact with
the DRE from both the AHR
and ARNT
are known as the alpha-1 helix arms. Both helix arm extensions are
made up of positively charged amino acids that interact with the
target DRE negatively charged phosphate backbone, increasing the
stability of the interaction and processivity of target gene
expression.
Recognition of the DRE by the AHR-ARNT
heterodimer from similar response elements, such as the hypoxia
response element (HRE) which differs by only one nucleotide, is
crucial for allowing the proper gene expression by AHR.
Arginine 39 (R39)
of the AHR�s
alpha-1 helix specifically aids in recognition of the proper
response element by forming three hydrogen bonds with the two base
moieties in the DRE: GC and CG. Arginine�s two proton donors on the
NH2 residue end forms two hydrogen bonds with the guanine base
oxygen and nitrogen proton acceptors. The one proton donor on the
double bonded NH of the residue end forms one hydrogen bond with the
guanine base oxygen proton acceptor. In the HRE, the single
nucleotide difference from the DRE (G->A) allows for the absence
of one of the three hydrogen bonds formed by AHR�s
R39 in the DRE, allowing for the specific recognition of the DRE.
Along with R39, other polar residue hydrogen bonds and salt bridge
interactions on the alpha helices within the AHR-ARNT
heterodimer are needed for proper DRE binding. These interactions
occur within the DRE and nearby non-target DNA to efficiently bind
the heterodimer to DNA, which include Arginines 38 and 39 and
Lysines 62 and 65 in AHR.
As well as Histidine 94, Arginines 91, 101 and 102, and Serine 91 in
ARNT.
It should be noted that there is an inconsistency between the PDB
file and Seung-Hyeon et al. 2017 where the PDB file shows Lysine 62
binding to an Adenine, the paper shows it bound to Thymine. In
addition, there should be a salt bridge interaction between Lysine
128 of the ARNT
bHLH at the same point though this interaction does not seem to take
place in the PDB.
V. References
Seung-Hyeon Seok, Woojong Lee, Li Jiang,
Kaivalya Molugu, Aiping Zheng, Yitong Li, Sanghyun Park,
Christopher A. Bradfield, and Yongna Xing. (2017). Structural
hierarchy controlling dimerization and target DNA recognition in
the AHR transcriptional complex. PNAS, Volume 114, Issue 21,
Pages 5431-5436. https://www.pnas.org/content/114/21/5431
Junsei Mimura and Yoshiaki Fujii-Kuriyama.
(2003). Functional role of AhR in the expression of toxic
effects by TCDD. Biochimica et Biophysica Acta (BBA)-General
Subjects, Volume 1619, Issue 3, Pages 263-268.
https://www.sciencedirect.com/science/article/pii/S0304416502004853
Brad R. Evans, Sibel I. Karchner, Lenka L.
Allan, Richard S. Pollenz, Robert L. Tanguay, Matthew J. Jenny,
David H. Sherr and Mark E. Hahn. (2008). Repression of Aryl
Hydrocarbon Receptor (AHR) Signaling by AHR Repressor: Role of
DNA Binding and Competition for AHR Nuclear Translocator.
Molecular Pharmacology, Volume 73, Issue 2, Pages 387-398.
http://molpharm.aspetjournals.org/content/73/2/387
Cornelia Dietrich and Bernd Kaina. (2010).
The aryl hydrocarbon receptor (AhR) in the regulation of
cell�cell contact and tumor growth. Carcinogenesis, Volume 31,
Issue 8, Pages 1319�1328. https://doi.org/10.1093/carcin/bgq028
Konkel, A. and Schunck, W.H. (2011). Role of
cytochrome P450 enzymes in the bioactivation of polyunsaturated
fatty acids. Biochimica et Biophysica Acta (BBA)-Proteins and
Proteomics, Volume 1814. Issue 1, Pages 210-222.
https://www.sciencedirect.com/science/article/pii/S157096391000258X
Back to Top