IkBb/NF-kB p65 Homodimer
Complex
Joe Duke-Cohan '22 and Andrew Savidge '21
Contents:
I. Introduction
The nuclear factor-kB (NF-kB) family of eukaryotic
transcription factors (TFs) are collectively involved in effecting
change at both the organismal and cellular levels through regulation
of gene expression related to immune and inflammatory responses as
well as cellular growth and apoptosis, respectively (Kumar et al.,
2004). The critical nature of the genes over which NF-kB proteins
exert control within these roles is evident from the disorders
arising from abnormal NF-kB constitutive activation, including
general immune diseases such as arthritis, inflammatory bowel
disease, and inflammatory lung disease as well diverse cellular
dysregulatory conditions (cancers) from leukemia and lymphoma to
neuroblastomas and glioblastomas (Okamoto, 2006; Atreya et al.,
2008; Park & Christman, 2006; Herwamana et al., 2008; Staudt,
2000; Widera et al., 2007; Smith et al., 2007).
The NF-kB TFs are related structurally by a ~300 residue homologous
peptide sequence (a majority of their structure), the Rel homology
region (RHR), and by their shared activity as homo- and heterodimers
whose integrative diversity contributes to extensively variable
DNA-binding capacity (Mosialos & Gilmore, 1993). Furthermore,
NF-kB TFs are functionally related by interactions with IkB proteins
that inhibit NF-kB nuclear localization and DNA-binding, relegating
the resulting IkB-NF-kB complexes to the cytoplasm. One of the more
common complexes is that formed between IkBb and the p65 homodimer.
The ligand-dependent ubiquitination and phosphorylation of the
former within the cytoplasm leads to its degradation and the release
of the p65 homodimer to the nucleus where it takes effect (Fig. 1).
Though, as Malek et al. (2003) show, hypophosphorylated IkBb-p65
homodimer complex may also migrate as a whole into the nucleus and
bind DNA in a stable complex. Phosphorylation and acetylation of the
latter also contributes to its DNA-binding capacity.
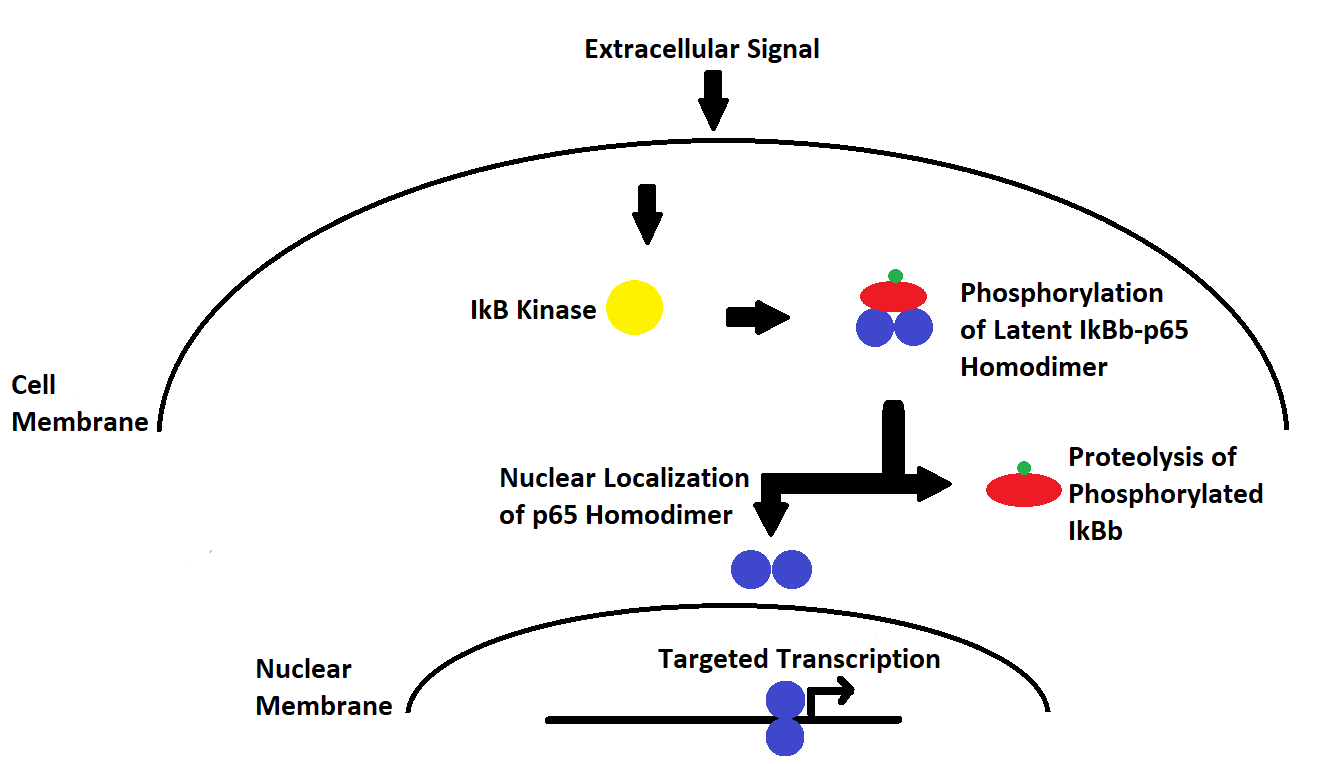
Figure 1. Annotated pathway of induction of IkBb degradation and
release of p65 homodimer to localize to the nucleus and effect
transcriptional change. Red oval represents IkBb. Blue circles
represent p65 TF subunits. Green circle represents phosphate group(s).
II. General Structure
The IkBb/NF-kB p65 homodimer complex is comprised of one 359
residue IkBb
, 38.04 kDa in weight, bound to two dimerized NF-kB p65 TF
, both 273 residues and 30.97 kDa in weight (note that the full p65
subunit chains are not depicted in complex with IkBb because their
structures could not be fully ascertained in complex by Malek et al.
(2003); the full structures are, however, depicted bound as a dimer
to DNA).
The IkBb inhibitor is comprised of 6
, each consisting of two antiparallel a-helices linked by a
sharp loop. In turn, each repeat is followed on either end by a
short B-hairpin turn whose 180-degree turn retains the parallel
orientation of the repeat domain. Hydrophobic stacking between the
helices of the ankyrin repeats stabilizes the extended structure of
IkBb. Each p65 subunit has a bipartite structure consisting of an
N-terminal RHR and a C-terminal transactivation domain (TAD)
separated by a nuclear localization signal (NLS)
. The RHR is folded into two immunoglobulin-like domains that
contribute to DNA-binding specificity while the TAD contributes to
dimerization stability (*Chen et al., 1998) through a selection of
hydrophobic and polar contacts: Cys 197, Asn 200, Phe 213, Leu 215, His 245, Val 248, Ala 249 and
Val 251 form a at the dimer interface. Arg 198, Cys 216, Asp 217, Asp 243,
His 245 and Arg 246 make
.
Linkage between IkBb and the p65 homodimer is mediated by
interactions between the first two ankyrin repeats of IkBb and the
NLS domain of p65 subunit A, as well as contacts made between the
last three ankyrin repeats of IkBb and the dimerization domain of
the p65 subunits
. Within the NLS domain, sandwiched stacking between Phe73
and Phe76 of IkBb and Phe318 (Phe318 not pictured) of p65 subunit A
provides particularly stability among other
(as well as salt bridge and polar contacts). 'Tis important
to note that the minimal extended surface contact between IkBb and
the p65 homodimer and the resultant reliance on individual single
and sparse interactions is largely due to the bent structure of the
ankyrin repeat domain and its limited shared surface area with the
planar dimerization surface of the two p65 subunits. Nonetheless, in
total, ~4000 Å2 of the surface area of the IkBb/NF-kB p65 homodimer
complex - roughly half of its total surface area is buried (Malek et
al., 2003).
III. Signaling
Signaling is essential in regulating the activity of both the
IkBb/NF-kB p65 homodimer complex as a whole, as well as the p65
homodimer during its functioning as a transcription factor after
release form the IkBb inhibitor. The most immediately effective
regulatory step in control of the complex is the signal for
degradation of the IkBb inhibitor and the subsequent release of the
p65 homodimer to localize to the nucleus. This step is primarily
controlled by phosphorylation of selective residues such as Ser32
and Ser36 and polyubiquitination of Lys9 within a signal response
region of IkBb (overlapping the NLS therein as well) (Fig. 2).
Phosphorylation of all IkB proteins occurs via three pathways
involving kinase cascades. The canonical pathway is that by which
pro-inflammatory cytokines recruit adaptor proteins to the
cytoplasmic membrane where they, in turn, recruit IkB-kinase (IKK)
complex to phosphorylate IkB. IkB is then ubiquitinated and degraded
by protease activity, releasing the attached NF-kB dimer whose NLSs
are now available to signal its travel to the nucleus where it can
bind DNA and act in conjugation with other TFs to activate targeted
transcription (Viatour et al., 2005). The release of the dimer is
facilitated by the fact that IkB, as aforementioned, makes minimal
and localized contact with the p65 homodimer, so degradation of IkBb
affects release faster than would a protein with weaker but more
extensive hydrophobic surface contacts.
Chemical modification of the p65 homodimer affects its later
interactions at transcription sites. For example, phosphorylation of
on either p65 subunit has the potential to change the dimer’s
interactions with cofactors, variably determining the gene set it
can coactivate. Acetylation of the p65 subunits also affects their
activational capacity, specifically, acetylation of
and Lys310 (Lys310 not pictured). Acetylation of Lys221
enhances p65 homodimer DNA binding, potentially by impairing
interactions with IkBb. Acetylation of Lys310 is required for
effective transcriptional activation, though not for DNA binding,
potentially indicative of it being required for binding to cofactors
(Chen et al., 2004).
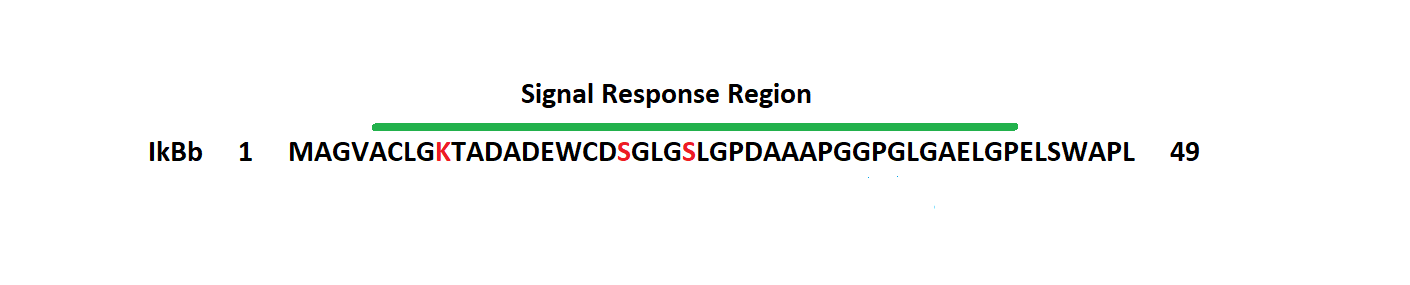
Figure 2. Annotated peptide sequence of IkBb (residues 1-49)
containing the signal response region (residues 5-42).
Polyubiquination target lysine and phosphorylation target serines
are all marked in red.
IV. DNA Binding
As aforementioned, the combinatiorial diversity of the various NF-kB transcription factors facilitates their variable DNA binding capacity. Specifically, the p65 homodimer binds a 10 bp 5’ NGGRNTTTCC 3’ (5’ GGAAANYCCN
3’ reverse complementary) consensus sequence by a mixture of van
der Waals and polar interactions nonspecifically to the DNA
sugar-phosphate backbone and with sequence specificity to bases
within the helix (**Chen et al., 1998). Both subunit A and subunit
B of the p65 homodimer have van der Waals contacts on the backbone
with Pro189 on both subunits interacting with phosphates linked to
antiparallel adenine nucleotides
. Additionally, Tyr36 on both subunits intercalates between
pairs of thymines on each strand with the base of each side chain
having polar interactions with the nearby phosphates as well.
Though both subunit A and subunit B retain polar interactions
with the backbone, only subunit A makes polar bonds to bases with
sequence specificity
. Besides the aforementioned thymine intercalation, Arg187
donates a hydrogen bond to O4 of the more terminal thymine
contacted by Tyr36. Arg35 hydrogen bonds O6 and N7 of the guanine
two bases terminal to Tyr36, while Arg33 hydrogen bonds O6 and N7
of the guanine one base terminal to Tyr36, and Glu39 hydrogen bonds N4 of the
cytosine immediately terminal to Tyr36.
V References
Kumar, A. et al. (2004). Nuclear
factor-kappaB: its role in health and disease. Journal of
molecular medicine, 82(7): 434-448.
Okamoto, T. (2006). NF-kappaB and
rheumatic diseases. Endocrine, metabolic and immune
disorders drug targets, 6(4): 359-372.
Atreya, I. et al. (2008). NF-kappaB in
inflammatory bowel disease. Journal of internal medicine,
263(6): 591-596.
Park, G.Y. & Christman, J.W. (2006).
Nuclear factor kappa B is a promising therapeutic target in
inflammatory lung disease. Current drug targets, 7(6):
661-668.
Herwamana, S. et al. (2008). The
NF-kappaB subunit Rel A is associated with in vitro survival
and clinical disease progression in chronic lymphocytic
leukemia and represents a promising therapeutic target. Blood,
119(9): 4681-4689.
Staudt, L.M. (2000). The molecular and
cellular origins of Hodgkin’s disease. The journal of
experimental medicine, 191(2): 207-212.
Widera, D. et al. (2007). Neural stem
cells, inflammation and NF-kappaB: basic principle of
maintenance and repair or origin of brain tumours? Journal
of cellular and molecular medicine, 12(2): 459-470.
Smith, D. et al. (2008). NF-kappaB
controls growth of glioblastomas/astrocytomas. Molecular
and cellular biochemistry, 307(1-2): 141-147.
Mosialos, G., & Gilmore, T.D. (1993).
v-Rel and c-Rel are differentially affected by mutations at a
consensus protein kinase recognition sequence. Oncogene,
8(3): 721-730.
Malek, S. et al. (2003). X-ray crystal
structure of an IkBb/NF-kB p65 homodimer complex. Journal
of biological chemistry, 278: 23094-23100.
*Chen, F.E. et al. (1998). Crystal
structure of p50/65 heterodimer of transcription factor NF-kB
bound to DNA. Nature, 391: 410-413.
Viatour, P. et al. (2005).
Phosphorylation of NF-kB and IkB proteins: implications in
cancer and inflammation. Trends in biochemical sciences,
40(1): 43-52.
Chen, L.F. et al. (2004). Shaping the
nuclear action of NF-kB. Nature reviews molecular cell
biology, 5: 392-401.
**Chen, Y.Q. et al. (1998). A novel DNA
recognition mode by the NF-kB p65 homodimer. Nature,
5(1): 67-73.
Back to Top