Homo Sapiens SPRY
Domain of RNA Helicase DDX1
Yangyang Liu '23 and Paige Milhon '23
Contents:
I. Introduction
The Homo sapiens RNA helicase DEAD-box protein 1 (DDX1)
is found within superfamily 2 of nucleic acid helicases. Human
ATP-dependent DDX1 protein is mainly involved in RNA processing by
unwinding both RNA-RNA and RNA-DNA duplexes and nuclease activity on
5' single strand RNA overhang. DDX1 has also been found to be
hijacked as a host factor in several viral replication pathways,
with HIV-1 mRNA via Rev-CRM1 pathway being the most well-known. The
most recent discovery is the interaction between DDX1 and
Coronavirus nonstructural protein 14 (nsp14), which enhances its
viral replication. Other than its involvement as a viral replication
factor, DDX1 is also overexpressed in tumor cells, having been found
to be associated with the formation and progression of germ-cell
tumours.
The DEAD-box family is characterized by their conserved DEAD sequence
motif and structurally conserved core. DDX1, on the other hand, shows
a unique structural feature of a large SPRY insertion domain in the
core of the helicase. The SPRY domain of human DDX1 (hDSPRY) appears
twice per asymmetric unit, termed
and
. These chains are
almost identical, differing only in four residues at the C-terminus.
Each hDSPRY acquires a compact β-sandwich conformation. Two layers of
β sheets stack together (β-sheet 1 and
β-sheet 2), and a third small β-sheet
covers one side of the sandwich (β-sheet
3).
The SPRY-domains are known to function as protein-protein
interaction platforms and also serve to recruit DDX1 to various
protein complexes.
II. General Structure
The hDSPRY is made up of amino acids 86-279 with one α/β
domain and is composed of three β-sheet layers:
β-sheet 1 is composed of
,
β-sheet 2 is composed of
, and
small β-sheet 3 is only composed of
. All of the β
strands are arranged in an antiparallel configuration, except for
two strands that are oriented parallel: β-16
and β-1 of β-sheet 1. β-16 of β-sheet 1
is only observed in chain B as it consists of
which do
not belong to the core of the SPRY domain and makes it a special
chain B feature. The residues form hydrogen bonds with strand 1 and
form an additional module which could be a crystal packing artifact.
Other than the special feature of β-16
in chain B, chain A’s C-terminal loop also adopts a conformation
that is totally different from chain B and the SPRY domain.
The long loop regions of each hDSPRY domain are mainly
located on one side of the β-sandwich.
(residues 150-164) is
situated between strands β-7 and β-8 of β-sheet 1 and covers a
hydrophobic patch on the sandwich’s concave side. This long loop is
common among structures of other SPRY domains. A similar observation
lies within the connecting loop between β-14 and 15 on the convex
side of β-sandwich, this loop shields the hydrophobic patch of
β-sheet 2 and has been termed ‘lid’ in RyR SPRY Structures (Figure
1).
Figure 1. Detail of the RyR1
F-loop (orange), indicating its hydrophobic side chains in green
sticks. These interact extensively with hydrophobic residues on the
rest of the SPRY2 domain (purple sticks). This is an example of the
hydrophobic interactions within a SPRY2 domain that is within the
same SPRY family as our structure. (Lau & Van Petegem, 2014)
III. Interface Between β-Sheet Layers
The structural integrity of the SPRY domain is maintained
in part by the residues at the interface of the two β-sheets. The
β-sheet 1 strands encompass an
intramolecular hydrophobic core with the opposite β-sheet
2 strands
. β 2, 6, 7, 8,
11, 12, 13, and 14 each have hydrophobic residues that stack
together through
.
Additionally, hydrogen bonds and salt bridges are present between
the two sheets and contribute to the overall conformational
rigidity (all amino acid involved in the interactions can be
viewed within the structure
). The amide
and carbonyl of Gly-148 are at a
hydrogen bonding distance from the O atom of
Tyr-135 (3.5/2.8 Å) and the amide group of
Lys-173 is at a hydrogen bonding distance from the
carbonyl of Ala-215 (2.6 Å). The
thiol groups of Cys139 and Cys145
conform such that the distance between their S atoms is
only 4.3 Å. Despite this proximity, there is no evidence that a
disulfide bond exists between them. These hydrogen and disulfide
bonds along with the salt bridges that contribute to the overall
rigidity can be explored in Table 1.
Table 1. Interactions within the
β-sandwich fold.
Amino
Acids
|
Interplanar
Distance (A)
|
Interaction
|
View
|
Gly-148 and Tyr-135 |
3.5/2.8
|
H-bond distance
|
|
Lys-173 and Ala-215 |
2.6
|
H-bond distance
|
|
Arg-146
and Asp-157
|
2.7
|
Salt bridge
|
|
Glu-184 and Lys-207
|
3.5
|
Salt bridge
|
|
Cys-139 and
Cys-145
|
4.3
|
No expected disulfide bond
|
|
IV. Conserved Hydrophobic Core and Positively Charged
Patch of hDSPRY
So far, there are only limited numbers of SPRY domains that
have been structurally characterized from eukaryotic proteins and
most of them are highly conserved. Within the highly conserved
hydrophobic core residues of hDSPRY, the residues that make up the
hydrophobic core to stabilize the β-sandwich fold in hDSPRY have
been found to be either conserved or substituted with similar
hydrophobic residues. The residues of most β-strands are conserved,
except for
at the
C-terminus: β-strand 15 of
β-sheet 3 and the potentially artificial β-strand
16 of β-sheet 1 in chain B. The degree of conservation
varies between the two sheets, with residues of β-sheet
1 being virtually identical to those of DDX1 orthologues
and residues of β-sheet 2, specifically
β-strands 2, 12, and 14, being less conserved (Figure 2).
Aside from the hydrophobic core, a highly conserved surface
patch formed by conserved residues of β-sheet
1, adjacent loop regions, and the N-terminal region of loop
D has been identified in hDSPRY. The accessibility of the surface
patch leads to the question of whether or not this conserved patch
might be a protein-protein interaction platform in hDSPRY. The
electrostatic surface potential of this patch is positively charged,
which leads to the possibility of hDSPRY establishing
protein-protein interactions through electrostatic interactions. In
other SPRY domains, it has been found that loops mediate the
protein-protein interactions.
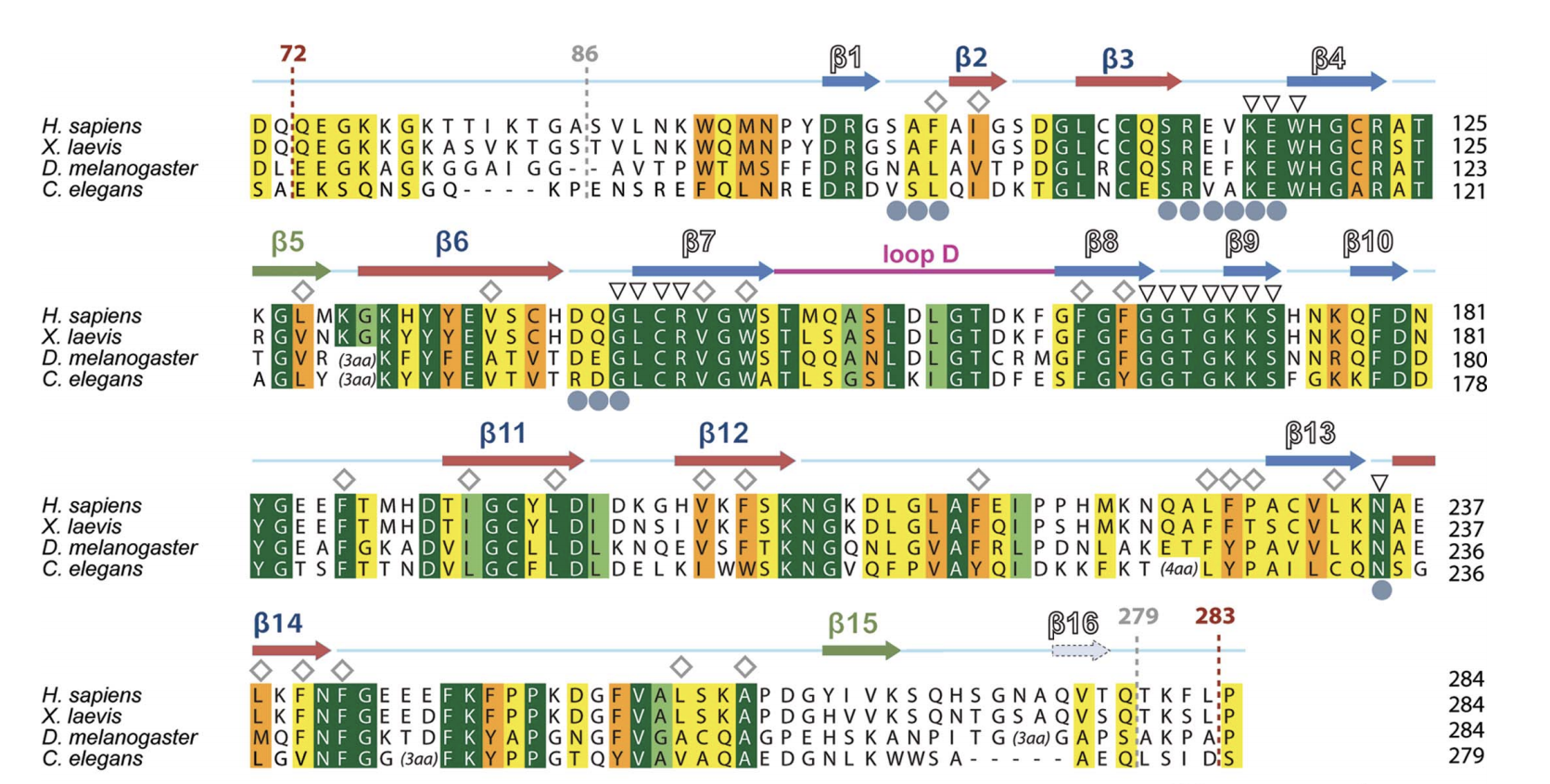
Figure 2. Sequence alignment of
hDSPRY with the SPRY domains of DDX1 homologues from other
eukaryotes. Dark green represents
regions of high conservation, light green
and orange represent regions of medium
conservation, and yellow represents
regions of low conservation. Residues of the hydrophobic core are
indicated by diamonds, residues of surface A are indicated by grey
circles, and residues of the conserved, positively charged surface
patch are indicated by triangles. Graphic on the right is the
sequence conservation mapped onto hDSPRY molecular surface (same
color codes are followed) (Kellner and Meinhart, 2015).
VI. SPRY Domain Structural Comparison
hDSPRY has been identified to have high structural similarity
to the SPRY domains of other eukaryotic proteins. The human
trithorax protein
(Chen et al., 2012)
and the previously mentioned mammalian
and
(Lau & Van
Petegem, 2014) being several of the proteins with the most
structural similarity with hDSPRY.
Whereas the core structures of hDSPRY and other SPRY domains
are structurally very similar, there are several major differences.
For instance, hDSPRY does not have extensive loop regions that are
comparable in length to the loop regions of the other domains. The longest
loop present in hDSPRY contains
and connects
β-strand 14 and β-strand
15 at the C-terminus. The Ash2L SPRY domain, on the other
hand, has a large 44-residue loop region that connects β-strands 11
and 12. Furthermore, the SPRY domain of Bre2, a homologue of Ash2L,
contains a 120-residue loop connecting β-strands 11 and 12. This loop
connecting β-strand 11 and β-strand
12 in hDSPRY, however, is only made up of
.
On top of differing in loop regions, hDSPRY and other SPRY
domains also differ in that the N- and C-terminus in hDSPRY never
directly interact. In the Ash2L SPRY domain, the C-terminus comes
together with the N-terminus to form a small β-sheet that extends
away from the β-sandwich and is not part of the SPRY domain. The N-
and C- terminus of hDSPRY are in close proximity, but it remains
unclear how they could form a linker that connects the inserted SPRY
domain to the core fold of DDX1.
Figure 3. Structural comparison
of hDSPRY (green) with the SPRY domain of Ash2L (red; PDB entry
3toj; Chen et al., 2012) using the DALI server (Holm &
Rosenström, 2010). Regions that show most significant structural
differences are indicated by intense color shading and are marked by
grey circles. The 44-residue loop of
Ash2L that is not resolved in the crystal structures is marked with
a red circle. Loop
D is also shown here.
VII. References
D’Cruz, A. A., Babon, J. J., Norton, R. S.,
Nicola, N. A., and Nicholson, S. E. (2013). Structure and function
of the SPRY/B30.2 domain proteins involved in innate immunity. Protein
Sci, 22(1):1-10. doi: 10.1002/pro.2185.
Kellner, J. N. and Meinhart, A. (2015).
Structure of the SPRY domain of the human RNA helicase DDX1, a
putative interaction platform within a DEAD-box protein. Acta
Cryst, F71:1176-1188. doi: 10.1107/S2053230X15013709.
Lau,
K. and Van Petegem, F. (2014). Crystal structures of wild type and
disease mutant forms of the ryanodine receptor SPRY2 domain. Nat
Commun 5, 5397. doi: 10.1038/ncomms6397.
Xu, L., Khadijah, S., Fang, S., Wang, L., Tay,
F. P. L., and Liu, D. X. (2010). The Cellular RNA Helicase DDX1
Interacts with Coronavirus Nonstructural Protein 14 and Enhances
Viral Replication. Journal of Virology, 84(17):8571-8583.
doi: 10.1128/JVI.00392-10.
~ Back to Top ~